|
|
Abstract
Surface skin imaging has long been the only non-invasive way for skin
imaging reflecting only the image provided by the stratum corneum, however
in the past few decades, thanks to the technological development, sub-surface
skin imaging have been made possible providing non-invasive in depth cutaneous
images by means of dermoscopy, optical coherence tomography, cutaneous ultra-sonography
and Laser confocal microscopy. This review aims at providing insight into
each of these modalities.
Introduction
The attempt to improve the accuracy of the diagnosis of different skin
diseases, especially for melanocytic skin lesions, has led to the development
of noninvasive imaging tools, such as dermoscopy, optical coherent tomography,
in vivo reflectance mode confocal microscopy, specrophotometric intracutaneous
analysis, and high-frequency ultrasound. They use the multiple reflection
indices of different chromophores of the skin when exposed to light to provide
highly detailed image of skin lesions [1].
Nowadays, dermoscopy is widely used in the routine clinical practice while
the other techniques are used mainly for research purposes.
Optical coherence tomography
Optical coherence tomography (OCT) is a non-invasive technique for the
morphologic investigation of tissues. It is based on the reflection of light
waves by biological tissues in the near-infrared region. An optical fiber
projects a light beam onto the skin, creating two-dimensional cross sectional
images that are comparable with the non-invasive virtual biopsies [2].
The technique provides real-time skin images 2 or 3 dimensional to a depth
of 1.8 micro meter with a lateral resolution of 10-20 micro meter [3].
Recently, high-definition OCT (HD-OCT) scanners have been developed to provide
significantly higher lateral resolution (1-3 micro meter ) [4]
OCT is a promising tool for various dermatologic conditions, allowing
for the visualization of the stratum corneum, viable epidermis, papillary
dermis, and appendages such as hair follicles and sweat ducts [3]
OCT is an extremely active and dynamic area of research and draws heavily
from the rapidly developing technology base in photonics and lasers. Different
modified technologies were introduced to improve the image resolution. Polarization-sensitive
OCT has the ability to visualize and quantify the birefringence properties
of skin especially collagen. Loss of collagen structure and integrity is
often associated with abnormalities of the skin, including tumors and connective
tissue diseases, suggesting that birefringence assessments may prove valuable
as a diagnostic indicator of certain cutaneous pathologies [5].
OCT of normal skin [2]
The appearance of healthy images at different anatomic sites is of importance,
in particular when interpreting OCT images of pathological processes. Normally,
the stratum corneum is only visible on the palmoplantar skin. At these sites,
OCT images display a wavy surface due to the dermatoglyphics. The horny
layer of the palmoplantar skin is a well-defined, thick, homogenous, low-scattering
band showing some high-scattering sweat gland ducts inside [6].
The border between the cornified and living epidermis is usually distinct,
whereas the dermo-epidermal border is frequently blurred (Fig 1).
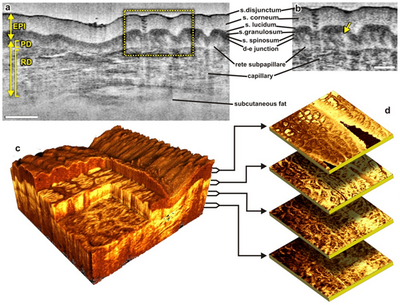 Fig 1:
Different ways to display micro-morphological information obtained
using OCT.
(a) B-scan of skin above the proximal interphalangeal joint of
the middle finger obtained using 1300 nm system.
(b) Magnified view of different sub-layers of epidermis and
dermis. The yellow arrow points toward the dermal-epidermal
junction.
(c) 3D rendering of the same region reconstructed from 1024
B-scans.
(d) En face sections of the same region separated in depth by
360 µm.
The scale bars in (a) denotes 500 µm and the scale bar in
(b) denotes 200 µm [6] |
|
Applications in dermatology:
- Skin tumors [7]
- Basal cell carcinoma: Basal cell nests show a characteristic
hypo-reflective border. OCT is used mainly for identification, diagnosis
of the type, evaluation of lateral borders, planning extent of excision
and follow-up (Fig 2) [8]
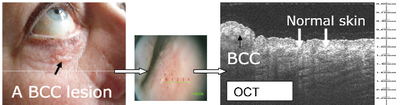
Fig 2: An example of a nodular BCC lesion, the black arrow points
at the lesion in the clinical photo and at the same lesion in the
OCT image. In between, the image from the OCT probe is seen with
a green line indicating where the OCT scan was performed. White
arrows indicate the adjacent normal skin in the OCT image [8]
|
|
- Squamous cell carcinoma in situ: actinic keratosis, Bowen's
disease, and erythroplasia of Queyrat show hyper-reflective keratin
depositions, acanthotic epidermis; irregular, but intact DEJ. OCT
is specifically important to rule out invasion.
- Invasive squamous cell carcinoma show hyper-reflective keratin
depositions, acanthotic epidermis and indistinguishable DEJ. OCT
can be used to evaluate the invasion depth and the efficacy of treatment.
- Malignant melanoma: show hyper-reflective structures extending
into the dermis and indistinguishable DEJ. OCT can be used to evaluate
the invasion depth and treatment efficacy.
- Other dermatological uses
- Hair analysis:
OCT can be used for in-vivo diagnosis of alopecia and follow-up.
Results are comparable to histology [9]
- Nail diagnosis:
Fungal elements are visualized as homogeneous, low-signal nail plate.
Currently there is no differentiation of pathogens but it can differentiate
it from inflammatory causes of nail changes such as lichen ruber,
psoriasis, atopic dermatitis [10]
- Dermatitis and psoriasis:
Non-invasive, reproducible follow-up, standardized measurement of
plaques (hyperkeratosis, acanthosis, papillomatosis). Development
of assessment scores is possible [11]
- Autoimmune diseases (e.g., lupus erythematosus):
Findings are comparable to histology [12]
- Blistering skin disorders:
Differentiation between intraepidermal and subepidermal blistering
is possible[13].
- Parasitosis:
OCT can be used for the rapid detection of parasitic pathogens such
as scabies and larva migrans [8]
- Measuring the skin when using topical treatments:
Assessment of skin atrophy (epidermis and dermis) in patients taking
corticosteroids [2]
In Vivo reflectance mode confocal microscopy
In vivo reflectance confocal microscopy (RCM) is a non-invasive imaging
tool that allows real-time visualization of cells and structures in living
skin with near histological resolution [14].
RCM is based on the collection of images from light reflected by living
tissue [15] . A confocal microscope consists
of a light source, a condenser, an objective lens and a detector, the light
source illuminates a small three dimensional spot within a sample, such
as skin. This illuminated spot is then imaged onto the detector through
a small aperture (pinhole). The small aperture allows only light that originates
from the focused illumination spot to be detected, whereas the light that
originates away from the spot is reflected [16].
Images are obtained in grey scale in which white represents total light
reflection and black represents no reflection at all. More light is reflected
when the tissue contains structures of size similar to the wavelength of
the light source (Fig 3) [17,18]
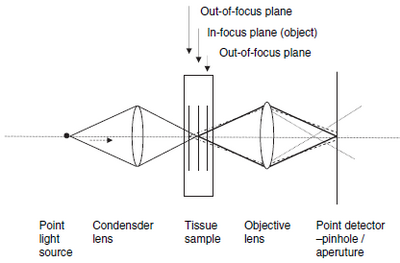 Fig
3: Diagram of a reflectance mode confocal microscope [18] |
|
Evaluation of normal skin
Imaging normal skin in real time usually takes place from the surface
and progressing deeper. Most superficial images correspond to the stratum
corneum. The stratum corneum produces the first image of the top surface
of the skin because of backscattered light at the water-to-stratum corneum
interface. Corneocytes are visualized as brilliant polygonal shapes of 10
to 30Mm size, and grouped in 'islands' separated by skin folds, which appear
very dark. The next layer is the stratum granulosum keratinocytes which
are 20 to 25Mm size and their nuclei show up as dark central oval structures
surrounded by a bright grainy cytoplasm. The stratum spinosum is located
20 to 100 micro meter deep. It consists of a tight 'honeycomb pattern' of keratinocytes
(10 to 15 micro meter size) with well-demarcated cell borders. Between 50 to 100
micro meter depths we can find the dermo-epidermal junction. Basal keratinocytes
are small (7-15 micro meter ) and bright [19], due
mostly to the presence of melanin inside the cell. The melanin in basal
keratinocytes is typically arranged in a supranuclear position.
Dermal papillae are observed as dark round areas surrounded by rings
of bright circles of basal cells containing highly reflective melanin granules.
Capillary loops are located in the centre of dermal papillae as black holes,
often showing bright erythrocytes rolling within them. Below the dermo-epidermal
junction, a network of collagen fibers and bundles (1Mm and 5 to 25
micro meter diameter,
respectively) can be observed within the papillary dermis and superficial
reticular dermis. Eccrine ducts appear as bright central hollow structures
that spiral through the epidermis and dermis. Hair shafts with pilosebaceous
units can be observed as central hollow structures with elliptical elongated
cells at the circumference, with a central white structure corresponding
to the hair shaft (Fig 4) [19]
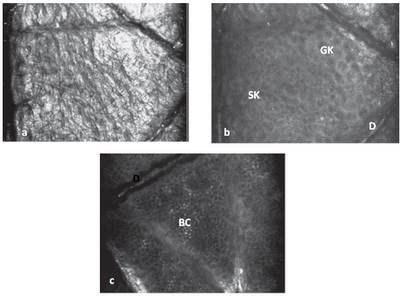 Fig
4: a) Confocal image of stratum corneum level showing high
refractivity. b) Slightly oblique image showing the presence
of granular keratinocytes (GK) and spinous keratinocytes (SK). c)
Confocal image at dermo-epidermal level (D) showing basal cell
layer (BC) [18] |
|
Use of RCM in clinical dermatology
- RCM findings of inflammatory skin conditions
- Contact dermatitis:
Features of contact dermatitis show spongiosis which appears as
intercellular brightness. The presence of epidermal inflammatory
cellular infiltrate can be visualized as bright round or oval structures
9 to 12 micro meter size interspersed between keratinocytes. Areas of necrotic
epidermis, perivascular inflammatory infiltrate, and increased size
and brightness of basal keratinocytes are also seen in both types
of reaction [20]
- Psoriasis:
The major features that distinguish the uninvolved skin from the
lesional psoriatic skin are increased numbers of dermal papillae
with enlarged dermal blood vessels inside them to supply the proliferative
lesion with circulating erythrocytes [21].
Other features are parakeratosis, clusters of polymorph-nuclear
leucocytes forming the Munro's microabscesses visualized as highly
refractile compared with the surrounding keratinized background
and thinning of the granular layer [22].
- Rosacea:
RCM histopathology reveals increased diameters of the pilosebaceous
ducts, tortuous capillaries and a characteristic perifollicular
and perivascular inflammatory infiltrate [23].
- RCM findings of cutaneous infections
- Fungal infections:
RCM enables rapid real-time identification of branched hyphae, visualized
as a network of long, dark, sometimes septated structures [24].
- Bacterial folliculitis:
Folliculitis imaged with RCM can be diagnosed by direct observation
of a hair follicle surrounded by a significant number of small bright
granular cells (neutrophils), which can be also found within the
subcorneal pustules. In addition, severe spongiotic epidermis and
capillary dilatation in the dermal papillae can be observed [23].
- Viral infections:
Warts imaged with RCM show hyperkeratotic stratum corneum and presence
of multiple highly refractile round structures measuring 20 to 40
micro meter size within the lesion.
- Cutaneous herpes infections:
Their main features are the presence of pleomorphic big round cells
with dark cytoplasm, identified as ballooned keratinocytes, and
internal round bright structures corresponding to multinucleated
giant cells.
- RCM findings of skin neoplasms
- Melanocytic nevi:
RCM of nevi show round to oval bright refractive cells with centrally
positioned dark round nuclei [25].
Dermal papillae are uniformly distributed and circumscribed by a
rim of refractive monomorphous cells (edge papillae) that correspond
to small melanocytes and melanin-rich keratinocytes, without any
cytological atypia. In junctional nevi, melanocytes are at the dermo-epidermal
junction level [26]. On the contrary,
in compound and dermal nevi, they are seen within the papillary
and reticular dermis, near the vessels. Sporadically, small brilliant
dendrites in the epidermis can be observed [26]
.
- Melanoma:
Confocal features suspicious for melanoma include structural changes
in the spinous and granular layers, keratinocyte disarrangement,
and loss of intercellular demarcation (disruption of the 'honeycomb
pattern') [25]. Enlarged atypical
cells with pleomorphic morphology, variable refractivity and angular
nuclei may be found in several layers of the epidermis (pagetoid
dissemination), and in the dermis. A useful advantage of RCM is
that it enables identification of abnormal intra-epidermal melanocytic
proliferation, granules, and dendritic structures in clinically
amelanotic melanomas (Fig 5) [27].
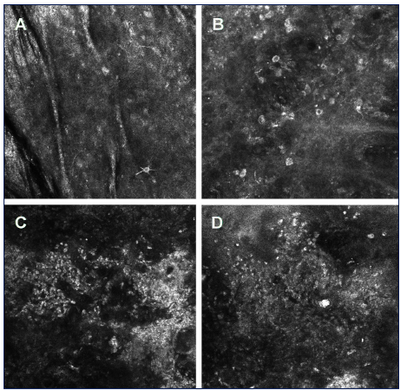 Fig
5: Reflectance confocal microscopy of melanoma. A - melanoma in
situ with the presence of a few dendritic cells in superficial
layers of the epidermis. B - melanoma in situ with the presence of
numerous atypical pigmented cells and disarray of the epidermis. C,
D - melanoma 3 micro meter Breslow thickness (C) and 1,3 micro
meter Breslow thickness
(D) with the total disorganization of the epidermis structure and
the presence of polymorphonuclear bright cells [1]
|
|
- Actinic keratosis:
The main features of actinic keratosis assessed by in vivo RCM include
irregular hyperkeratosis in the stratum corneum. In addition, the
stratum granulosum is almost identical to that of normal skin, with
dark nuclei, contrasted against the bright refractile cytoplasm
of the keratinocytes. Whereas the nuclei in the stratum spinosum
and stratum basale vary in shape, size, and haphazard orientation,
these findings correspond to nuclear enlargement with pleomorphism
in a pattern consistent with architectural disarray, which does
not involve the full thickness of the epidermis [28].
- Squamous cell carcinoma:
Confocal features suggestive of SCC are full thickness architectural
disarray and nuclear enlargement with pleomorphism observed from
the basal layer to the stratum granulosum.
- Basal cell carcinoma:
Reflectance confocal microscopy morphological characteristics of
basal cell carcinoma include: the presence of pleomorphism and architectural
disorder of the overlying epidermis, indicative of actinic damage
or the presence of the tumour, the presence of islands of refractive
tumour cells with elongated monomorphic basaloid nuclei, associated
with intervening areas of low refractility, which might correspond
to the mucinous stroma, nuclei of tumour cells that are polarized
along the same axis of orientation, disrupting the normal honeycomb
pattern of the epidermis and the dermal papillae architecture, increased
dermal vasculature with prominent dilatation and tortuosity of blood
vessels and trafficking of leucocytes is easily identified as bright,
highly refractile round cells along the endothelial lining[29]
- Monitoring of the treatment response:
RCM is a useful tool in monitoring the response of actinic keratoses
[30] and BCC to topical treatment such
as imiquimod [31]
- RCM usefulness in cosmetics:
Reflectance confocal microscopy is a sensitive tool for the detection
of histological changes of the epidermis and papillary dermis because
of ageing. Changes in the epidermis and the superficial dermis following
the treatment with anti-ageing products, lasers and pulsed light therapies
can be monitored by RCM before, during and after application. RCM greatly
enhances the assessment of pigmentary changes in human or animal skin
over time and in response to specific stimuli such as ultraviolet radiation
exposure as well as in pre-evaluation of tattoos before laser removal,
helping to predict their clinical outcome [32].
Spectrophotometric intracutaneous analysis
Spectrophotometric intracutaneous analysis (SIA) is a skin-imaging technique
that allows the rapid, noninvasive in vivo quantification and assessment
of eumelanin, oxyhemoglobin, and dermal collagen within the human skin.
SIAscopy produces independent linear measurements of each of these chromophores
producing images called SIAscans. The technique uses light reflected from
the skin in the visible and infrared spectra to produce images of the epidermal
and dermal melanin, the vasculature, and the dermal collagen content within
the lesion. The interpretation of image colors allows estimations of the
underlying histopathologic features (Fig 6). The device is available
in contact and non-contact types [33,34].
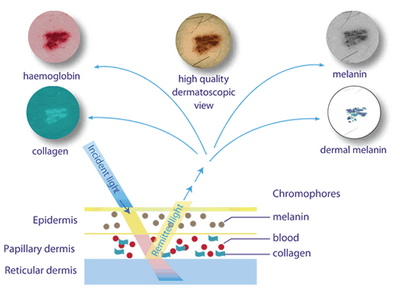 Fig
6: Colors of different skin chromophores observed by SIAscopy [34] |
|
SIAscopy applications in dermatology
1. Malignant melanoma Characteristic signs of invasive cutaneous melanoma observed by SIAscopy
include; the presence of melanin in the dermis indicating invasion into
the papillary dermis, collagen hole, and an area with no papillary collagen
present, indicating destruction or replacement of the papillary dermis
by melanoma. The blood distribution map shows the increase in blood
levels on the lesion periphery ("erythematous blush"), which is indicative
of inflammation and vasodilatation, often associated with invasive skin
tumors. In addition, there is a total lack of blood in the centre of
the lesion in the area which coincides with the dermal melanin, further
indicating destruction, or replacement of the papillary dermis. The
collagen map also shows fibrosis on the lesion periphery which is associated
with early, invasive melanoma (Fig 7) [35,36].
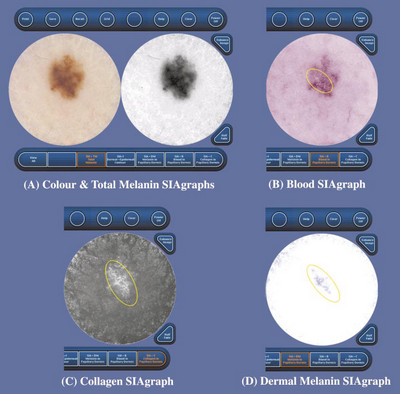
Fig 7: SIAgraphs of a superficial spreading melanoma. The
colour and total melanin SIAgraphs (A) are unremarkable. However,
the blood SIAgraph (B) shows a subtle blood displacement with
erythematous blush (circled). The collagen SIAgraph (C) shows no
holes as this is only a Clark's level II melanoma, although there
are large quantities of irregular collagen (circled) consistent with
fibrosis. The dermal melanin SIAgraph (D) shows dermal melanin
irregularly distributed across a large area of the lesion (circled)
[36] |
|
2 - Pigmented basal cell carcinoma SIAscopy is of little value in differentiating malignant melanoma from
pigmented basal cell carcinoma as both show the same features [36]
SIAscopy can be used in diagnosing psoriasis and eczema and assessment
of treatment regimen efficacy [37] including
phototherapy [38].
Other possible uses include assessment of aging skin [39],
acute burn depth [40] and linear scars
hypertrophy [41]
Cutaneous ultrasonography
Used since the 70s in dermatology, ultrasonography is based on the reflection
of sound waves throughout the tissues [42].
According to the anatomical structure, its vascularization, and density,
the ultrasound waves are reflected back to the transducer that converts
them into a gray scale, observed on the monitor [43].
The higher the frequency of the waves emitted by the transducer, the better
the spatial resolution and subsequent visualization of structures near it.
The introduction of transducers with frequency higher than 15 MHz produced
the high-frequency ultrasound (HFUS). The shortest wavelength obtained by
this frequency allowed a better assessment of superficial structures, significantly
expanding its use in cutaneous diseases [44].
For dermatologic purposes, the ultrasound scanners using high frequencies,
i.e. 15 MHz and more, producing a resolution of at least 50 µm, are essential.
For such ultrasound characteristics, the term high-frequency ultrasound
or high resolution ultrasound has been introduced [45].
During the propagation in the skin, the ultrasound waves undergo reflection,
retraction, scattering, attenuation or absorption by the examined structures,
mostly at the border of the adjacent media, generating various amplitudes
of echoes influencing the ultrasound image characteristics.
Normal ultrasonographic findings of the skin:
It shows a well-defined hyperechoic band known as epidermal "entry echo"
at the interface between the transducer and the skin. Underneath, the epidermis
is seen as hyperechoic layer with small hypoechoic areas corresponding to
hair follicles, vessels, and sebaceous glands. The next layer, the subcutaneous
tissue, is hypoechoic with hyperechoic connective tissue septa separating
the adipose lobules, more deeply, the superficial fascia covering the muscular
tissue can be seen as hyperechoic regular line (Fig 8) [46]
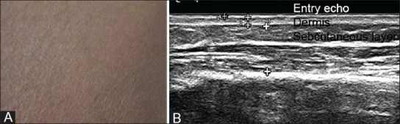 Fig
8: Ultrasound showing the normal layers of the skin [46]. |
|
The nail unit structure on HRUS shows superficial bilaminar hyperechoic
parallel lines representing dorsal and ventral plates and underlying hypoechoic
bed [47].
Cutaneous ultrasonography applications:
HRUS is used for the measurement of the thickness and the invasion depth,
assessment of the borders, and follow-up after surgery, cryotherapy, and
laser treatment for different benign and malignant skin tumors such as malignant
melanoma, basal cell carcinoma and seborrheic keratosis. Skin tumors present
as focal hypoechoic areas within the hyperechoic epidermis and dermis. Color
and power Doppler help to identify the vascularity in the lesions. Presence
of abnormal intra or peri-tumoral low resistance pulsatile flow signals
suggests the malignant nature of the lesion (Fig 9) [48].
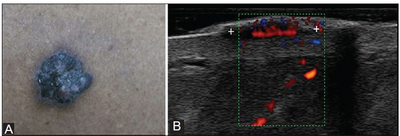 Fig
9: (A) Malignant melanoma seen as an elevated pigmented lesion
with irregular shape and borders. (B) HRUS shows
well-defined, solid, homogenously hypoechoic lesion in the dermis
with multiple vessels arising from the base, suggestive of high
vascular density [46] |
|
- Sclerodemal changes of skin
HRUS can be used to monitor the course and therapeutic efficacy of the
treatment of diseases e.g. morphea, systemic scleroderma and lipodermatosclerosis
[49].
- Chronic inflammatory dermatoses
- Psoriasis
HRUS examination shows thickened epidermis with hyperechoic as the
superficial scales produce a hyper-reflective epidermal band, and
a hypoechoic band of variable thickness may be seen in dermis in
the acute phase (Fig 10) [50].
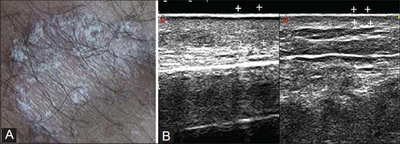 Fig 10:
Ultrasonography examination of psoriatic plaque showing thickened
hyperechoic epidermis and dermis compared to contralateral skin, as
the superficial scales produce a hyper-reflective epidermal band [50] |
|
- Contact dermatitis
HRUS of the affected areas in these cases shows significantly increased
thickness of dermis within a homogeneous echotexture and gross foci
of hypoechoic edema [51].
- Evaluation of exogenous component like foreign bodies and
cosmetic fillers in the skin:
The USG picture of foreign body is typical and consists of small, strong
reflector surrounded by hypoechoic tissue. The combination of this appearance
and positive clinical history is pathognomonic of the diagnosis [52].
On HRUS, hyaluronic acid and pure silicone are anechoic, while polyethylmethacrylate
and silicone oil, calcium hydroxyapatite are hyperechoic with variable posterior
acoustic shadowing [53].
- Evaluation of nail involvement in systemic diseases and nail
bed lesions e.g. glomus tumors, nail bed cysts, and subungual
exostosis [42].
- Evaluation of other dermatologic diseases [42]
- Allergic dermatitis
- Nodular erythema
- Dermatomyositis
- Sarcoidosis
- Lymphedema of the limbs
- Wound healing
- Follow-up of localized burn lesions.
Dermatoscopy
Dermoscopy is a non-invasive technique that allows a rapid and magnified
in vivo observation of the skin with the visualization of morphologic features
invisible to the naked eye. The optical principle involved in dermoscopy
is the interactions of light with the skin. The refractive index of the
stratum corneum is higher than that of air; much of the incident light is
reflected off the surface of the skin overwhelming the retina obscuring
the visualization of light that is reflected from the deeper layers of the
skin [54]. Two types of dermoscopy are
recognized; the non polarised type (NPD) in which a liquid interface is
used to eliminate the backscattering of light from the stratum corneum
(Fig 11) and polarised dermoscope (PD) in which two polarizers are
used to achieve cross-polarization allowing the dermoscope to capture the
backscattered light from the deeper layers of the skin (Fig 12).
The main advantages of the cross-polarized system are that it eliminates
the necessity of a liquid interface and it does not require direct contact
with the skin [55].
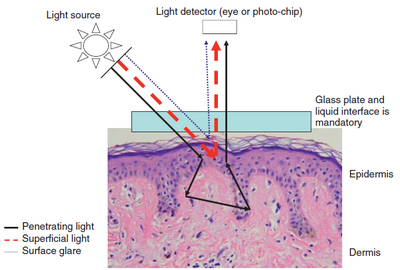 Fig
11: Schematic representation of optical properties of light
during the use of contact NPD with a liquid interface [55] |
|
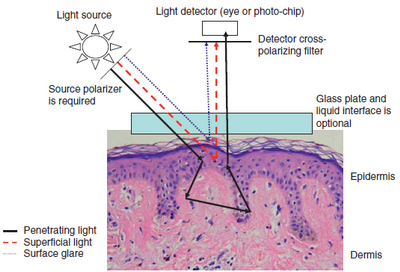 Fig 12:
Schematic representation of optical properties of light during the
use of PD [55] |
|
Dermatoscopy of normal skin
The structures and details of the normal skin vary depending upon the
skin site, skin phototype and the degree of photodamage. Once the features
of normal skin are recognized, the boundary between normality and pathology
can be better recognized (Fig 13) [56].
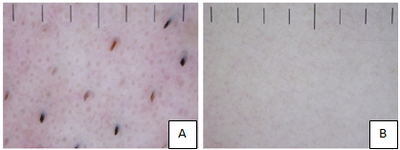 Fig 13:
A) Normal facial skin (male skin, phototype I), showing
dense follicular units.
B) Normal truncal skin (male skin, phototype I), showing an
absence of detail [56] |
|
Dermatoscopy applications [57]
- Classic dermoscopy: for the diagnosis of pigmented and non-pigmented
skin tumors including melanocytic and non-melanocytic and benign and
malignant skin tumors.
- Entomodermoscopy: for the diagnosis of skin infections and infestations
caused by parasites or viral, bacterial, fungal or protozoan infections.
- Inflammoscopy: for the diagnosis of inflammatory skin diseases such
as psoriasis, lichen ruber planus, pityriasis rosea and many others.
- Trichoscopy: for diagnosing hair and scalp disorders.
- Capillaroscopy: of the nail fold capillaries for the screening of
autoimmune diseases.
- Dermoscopy for treatment decision and monitoring: This application
gains importance especially in the light of the steadily increasing
availability and use of topical treatment options for non-melanoma skin
cancer.
References
1. Kardynal A, Olszewska M. Modern non-invasive diagnostic
techniques in the detection of early cutaneous melanoma. Journal of dermatological
case reports, 2014, 8(1): 1.
2. Welzel J. Optical coherence tomography in dermatology:
a review. Skin Res Technol 2001; 7: 1-9.
3. Pagnoni A, Knuette A, Welker P, et al. Optical coherence
tomography in dermatology. Skin Res Technol, 1999; 5: 83-87.
4. Gambichler T, Valavanis K, Plura I, et al. In vivo determination
of epidermal thickness using high definition optical coherence tomography.
Br J Dermatol 2014; 170: 737-739.
5. Applegate BE, Yang C, Rollins AM, Izatt JA. Polarization
resolved second-harmonic-generation optical coherence tomography in collagen.
Opt Lett 2004; 29:2252-4.
6. http://www.zmpbmt.meduniwien.ac.at
7. Schmitz L, Reinhold U, Bierhoff E, Dirschka T. Optical
coherence tomography: its role in daily dermatological practice. JDDG: Journal
der Deutschen Dermatologischen Gesellschaft, 2013; 11(6), 499-507.
8. Mogensen M, Thrane L, Jřrgensen T M, Andersen P E, Jemec
G B E. Optical coherence tomography for imaging of skin and skin diseases.
In Seminars in cutaneous medicine and surgery, 2009; September, Vol. 28,
No. 3, pp. 196-202. WB Saunders.
9. Bartels NG, Stieler K, Richter H, Patzelt A, Lademann
J, Blume-Peytavi U. Optical coherent tomography: promising in vivo measurement
of hair shaft cross section. Journal of biomedical optics, 2011; 16(9),
096003-096003.
10. Hoeller-Obrigkeit D, Abuzahra F, Spoeler F, Foerst
M, Brans R, Erdmann S, Merk H F. Optical coherence tomography as a non-invasive
diagnostic perspective for real time visualization of onychomycosis-a pilot
study. In Journal Der Deutschen Sermatologischen Gesellschaft 2009; November
(Vol. 7, No. 11, pp. 1014-1014). Commerce Place, 350 Main ST, Malden 02148,
MA USA: Wiley-Blackwell Publishing Inc.
11. Welzel J, Bruhns M, Wolff HH. Optical coherence tomography
in contact dermatitis and psoriasis. Archives of dermatological research,
2003; 295(2), 50-55.
12. Gambichler T, Hyun J, Moussa G, Tomi NS, Boms S, Altmeyer
P, Hoffman K, Kreuter A. Optical coherence tomography of cutaneous lupus
erythematosus correlates with histopathology. Lupus, 2007; 16(1), 35-38.
13. Mogensen M, Morsy HA, Nurnberg BM, Jemec GBE. "Optical
coherence tomography imaging of bullous diseases." Journal of the European
Academy of Dermatology and Venereology 22, no. 12 (2008): 1458-1464.
14. Markisz JA, Aquilia MG. Technical magnetic resonance
imaging. McGraw Hill Professional 1996.
15. Webb, R. H. Confocal optical microscopy. Reports on
Progress in Physics, 1996; 59(3), 427.
16. Rajadhyaksha M, González S, Zavislan JM. Detectability
of contrast agents for confocal reflectance imaging of skin and microcirculation.
Journal of biomedical optics, 2004; 9(2), 323-331.
17. Rajadhyaksha M, González S, Zavislan JM, Anderson RR,
Webb R H. In vivo confocal scanning laser microscopy of human skin II: Advances
in instrumentation and comparison with histology1. Journal of Investigative
Dermatology, 1999; 113(3), 293-303.
18. González S, Gilaberte-Calzada Y. In vivo reflectance-mode
confocal microscopy in clinical dermatology and cosmetology. International
journal of cosmetic science, 2008; 30(1), 1-17.
19. Rajadhyaksha M, Anderson R, Webb RH. Video-rate confocal
scanning laser microscope for imaging human tissues in vivo. Applied optics,
1999, 38(10), 2105-2115.
20. Swindells K, Burnett N, Rius-Diaz F, González E, Mihm
MC, González S. Reflectance confocal microscopy may differentiate acute
allergic and irritant contact dermatitis in vivo. Journal of the American
Academy of Dermatology, 2004, 50(2), 220-228.
21. González S, Rajadhyaksha M, Anderson RR. Non-invasive
(real-time) imaging of histologic margin of a proliferative skin lesion
in vivo. Journal of investigative dermatology, 1998; 111(3), 538-539.
22. González S, Rajadhyaksha M, Rubinstein G, Anderson
RR. Characterization of psoriasis in vivo by reflectance confocal microscopy.
Journal of medicine, 1998; 30(5-6), 337-356.
23. González S, Rajadhyaksha M, González-Serva A, White
WM, Anderson R. Confocal reflectance imaging of folliculitis in vivo: correlation
with routine histology. Journal of cutaneous pathology, 1999; 26(4), 201-205.
24. Markus R, Huzaira M, Anderson RR, González S. A Better
Potassium Hydroxide Preparation?: In Vivo Diagnosis of Tinea With Confocal
Microscopy. Archives of dermatology, 2001; 137(8), 1076-1078.
25. Langley RG, Rajadhyaksha M, Dwyer PJ, Sober A J, Flotte
TJ, Anderson RR. Confocal scanning laser microscopy of benign and malignant
melanocytic skin lesions in vivo. Journal of the American Academy of Dermatology,
2001; 45(3), 365-376.
26. Pellacani G, Cesinaro AM, Seidenari S. In vivo assessment
of melanocytic nests in nevi and melanomas by reflectance confocal microscopy.
Modern pathology, 2005; 18(4), 469-474.
27. Busam K J, Charles C, Lohmann CM, Marghoob A, Goldgeier
M, Halpern A C. Detection of intraepidermal malignant melanoma in vivo by
confocal scanning laser microscopy. Melanoma research, 2002; 12(4), 349-355.
28. Aghassi D, Anderson RR, González S. Confocal laser
microscopic imaging of actinic keratoses in vivo: a preliminary report.
Journal of the American Academy of Dermatology, 2000; 43(1), 42-48.
29. Nori S, Rius-Díaz F, Cuevas J, Goldgeier M, Jaen P,
Torres A, González S. Sensitivity and specificity of reflectance-mode confocal
microscopy for in vivo diagnosis of basal cell carcinoma: a multicenter
study. Journal of the American Academy of Dermatology, 2004; 51(6), 923-930.
30. Goldgeier M, Fox CA, Zavislan JM, Harris D, González
S. Noninvasive imaging, treatment, and microscopic confirmation of clearance
of basal cell carcinoma. Dermatologic surgery, 2003; 29(3), 205-210.
31. Torres A, Niemeyer A, Berkes B, Marra D, Schanbacher
C, González S, Owens M, Morgan B. 5% Imiquimod cream and reflectance-mode
confocal microscopy as adjunct modalities to Mohs micrographic surgery for
treatment of basal cell carcinoma. Dermatologic surgery, 2004; 30(12p1),
1462-1469.
32. Middelkamp-Hup MA, Park HY, Lee J, Gilchrest BA, González
S. Detection of UV-induced pigmentary and epidermal changes over time using
in vivo reflectance confocal microscopy. Journal of investigative dermatology,
2006; 126(2), 402-407.
33. Cotton SD. A non-invasive imaging system for assisting
in the diagnosis of malignant melanoma, 1998. (Doctoral dissertation, The
University of Birmingham).
34. www.medxhealth.com
35. Menzies SW, Crotty K A, Ingvar C, McCarthy W H. An
atlas of surface microscopy of pigmented skin lesions: dermoscopy 2003.
Roseville: McGraw-Hill.
36. Moncrieff M, Cotton S, Claridge E, Hall P. Spectrophotometric
intracutaneous analysis: a new technique for imaging pigmented skin lesions.
British Journal of Dermatology, 2002; 146(3), 448-457.
37. Novaković L, Hawk J. Spectrophotometric intracutaneous
analysis: a novel technique in the differential diagnosis of psoriasis and
eczema. BRITISH JOURNAL OF DERMATOLOGY-SUPPLEMENT, 2002; 147, 104-104.
38. Novaković L, Cotton S, Hawk JL. Spectrophotometric
intracutaneous analysis as an early non-invasive predictor of efficacy in
the phototherapy of psoriasis. Photodermatology, photoimmunology & photomedicine,
2009; 25(2), 81-85.
39. Callaghan TM, Wilhelm KP. A review of ageing and an
examination of clinical methods in the assessment of ageing skin. Part 2:
Clinical perspectives and clinical methods in the evaluation of ageing skin.
International journal of cosmetic science, 2008; 30(5), 323-332.
40. Tehrani H, Walls J, Price G, Cotton S, Sassoon EM,
Hall P N. A prospective comparison of spectrophotometric intracutaneous
analysis to clinical judgment in the diagnosis of nonmelanoma skin cancer.
Annals of plastic surgery, 2007; 58(2), 209-211.
41. Kaartinen IS, Välisuo PO, Bochko V, Alander JT, Kuokkanen
HO. How to assess scar hypertrophy-a comparison of subjective scales and
spectrocutometry: a new objective method. Wound Repair and Regeneration,
2011; 19(3), 316-323.
42. Kleinerman R, Whang T B, Bard R L, Marmur E S. Ultrasound
in dermatology: Principles and applications. Journal of the American Academy
of Dermatology, 2012, 67(3): 478-487.
43. Wortsman X. Common applications of dermatologic sonography.
Journal of Ultrasound in Medicine, 2012, 31(1): 97-111.
44. Crisan M, Crisan D, Sannino G, Lupsor M, Badea R, Amzica
F. Ultrasonographic staging of cutaneous malignant tumors: an ultrasonographic
depth index. Archives of dermatological research, 2013, 305(4), 305-313.
45. Zmudzinska M, Czarnecka-Operacz M, Silny W. Principles
of dermatologic ultrasound diagnostics. Acta Dermatovenerologica Croatica,
2008, 16(3), 0-0.
46. Mandava A., Ravuri P R, Konathan R. High-resolution
ultrasound imaging of cutaneous lesions. The Indian journal of radiology
& imaging, 2013, 23(3), 269.
47. Altmeyer P, Hoffmann K, Stücker M, Goertz S, El-Gammal
S. General phenomena of ultrasound in dermatology. Ultrasound in Dermatology.
Berlin, Springer, 1992: 55-79.
48. Szymańska E, Nowicki A, Mlosek K, Litniewski J, Lewandowski
M, Secomski W, Tymkiewicz R. Skin imaging with high frequency ultrasound-preliminary
results. European journal of ultrasound, 2000, 12(1): 9-16.
49. Hesselstrand R, Scheja A, Wildt M, Ĺkesson A. High-frequency
ultrasound of skin involvement in systemic sclerosis reflects oedema, extension
and severity in early disease. Rheumatology, 2008, 47(1): 84-87.
50. Cammarota T, Pinto F, Magliaro A, Sarno A. Current
uses of diagnostic high-frequency US in dermatology. European journal of
radiology, 1998, 27: S215-S223.
51. Schmid-Wendtner M H, Burgdorf W. Ultrasound scanning
in dermatology. Archives of dermatology, 2005, 141(2), 217-224.
52. Soudack M, Nachtigal A, Gaitini D. Clinically unsuspected
foreign bodies the importance of sonography. Journal of ultrasound in medicine,
2003, 22(12), 1381-1385.
53. Wortsman X, Wortsman J. Sonographic outcomes of cosmetic
procedures. American Journal of Roentgenology, 2011, 197(5), W910-W918.
54. Anderson R R, Parrish J A. The optics of human skin.
Journal of Investigative Dermatology, 1981; 77(1), 13-19.
55. Wang S Q, Marghoob A A, Scope A. Principles of dermoscopy
and dermoscopic equipment. An Atlas of Dermoscopy, 2012.
56. Bowling, J. Normal skin. (2012): Diagnostic dermoscopy:
the illustrated guide, first edition. Edited by Bowling, J., published by
Wiley-Blackwell, London. Chapter 1. Page: 8.
57. Zalaudek I, Lallas A, Moscarella E, Longo C, Soyer
H P, Argenziano G. The dermatologist's stethoscope-traditional and new application
of dermoscopy. Dermatol Pract Conc. 2013; 3 (2): 11.
© 2015 Egyptian Dermatology Online Journal
|